PDF of this article (16 MB)
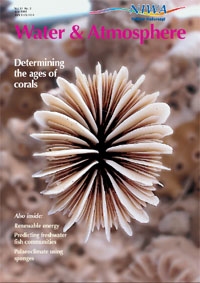
Michael Ellwood Michelle Kelly
Deep-sea sponges have the potential to provide unusually detailed information about ocean, and thus climate variations in the past.
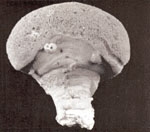

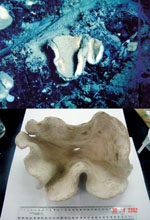


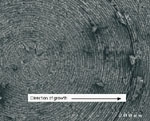
Marine sponges may seem unlikely candidates for providing information about past environmental conditions – but it is turning out that some species can do it very well! Scientists are interested in this information because in order to predict future changes in ocean chemistry it is essential to have records of past events as well as to understand what drives changes in ocean chemistry today. Because the ocean occupies about 70% of the earth’s surface, any change in ocean chemistry and physics is likely to impact on regional and global climate.
Information on how climate has changed in the past as a result of ocean variability is available from historical data, but this generally covers only very recent changes (up to a few hundred years). Longer-term ocean and climate information can be deduced from a range of historical markers – known as proxies – from which we can infer environmental conditions over time-scales of up to thousands of years. These so-called palaeocean and palaeoclimate proxies include oceanic sediment cores, ancient pollen, tree-rings, ice cores, glaciers and speleothems. (For examples of the use of some of these proxies see Water & Atmosphere 7(1): 14–16; 8(3) (“Fabulous foraminifera”); 9(1) (“Antarctic ice”); 9(4) (whole issue.)
Silica rings in sponges
Proxies provide crucial insights into past climate change and ocean variability. For a proxy to be really useful it needs to show up changes that happened over short time-spans and in specific areas. Also we need to know what time-span the proxy represents.
Certain marine sponges offer a unique approach to the problem of recording environmental change. These sponges and their spicules grow by putting on concentric layers of silica material on a seasonal basis, rather like tree rings. The concentrations of certain chemicals in the surrounding water, and the growth rate of the sponge itself, are archived in the silica deposited. The information in each layer of silica can be used to deduce environmental conditions on a range of scales: years, decades and centuries.
Most palaeoclimate work using sponges has used sclerosponges (see photos left). These grow extremely slowly and are relatively easily collected in tropical marine caves by SCUBA. For the world’s colder regions, however, other types of sponges need to be found.
Sponge discoveries
In recent research at NIWA in conjunction with Dr Bertrand Richer de Forges, Institut de Recherche pour le Development, Noumea, we have uncovered two “new” types of sponges that display numerous rings in their skeletons. In 1999, Michelle collected a large bowl-shaped rock sponge from the Norfolk Ridge in the Tasman Sea (left). X-ray analysis of a section from this specimen shows more than 140 distinct rings (shown below). When we analysed various seawater metals from the basal rings (oldest) to the outer edge rings (youngest) in the sponge, we found distinct fluctuations. Early analysis suggests that these fluctuations relate to past ENSO (El Niño/La Niña) climate events in the region. We are currently determining the exact age of this sponge using Silicon 32 (32Si) dating (see panel opposite page). We anticipate that the annual nature of the rings will be confirmed.
Another more recent discovery is a 1.4-m-long spicule made by a glass sponge (left) with about 440 very clear “tree-rings” visible in cross-section (left). Such long spicules are very rare and belong to the unusual glass-sponge genus Monoraphis. This spicule was collected by Dr Richer de Forges from the deep ocean off the Norfolk Ridge.
A powerful new tool
Our future research will focus on spicules from dead sponges deposited in deep-sea sediments, and land-based fossil spicules and sponges. By using a combination of sediment dating techniques, stratigraphic markers, and chemical analyses of spicules, we hope to reveal changes in ocean chemistry on millennium timescales (0–10,000 years and 10,000–100,000 years).
Global climate change is of extreme national and international significance and is linked to increases in atmospheric greenhouse gas concentration. Most scientists agree that climatic impacts of events such as the El Niño and La Niña will intensify with time, but the magnitude and extent are not known. The examination of sponge silica and the chemical signatures that they contain from the past and present will provide a powerful new tool for international efforts in reconstruction of past climate variability.
Teachers: this article can be used for NCEA Achievement Standards in Science (2.5), Chemistry, Biology and Physics (1.2), Biology (2.6, 3.4), Geography (3.6). See other curriculum connections at www.niwa.co.nz/pubs/wa/resources
Michael Ellwood is based at NIWA in Hamilton and Michelle Kelly is based at NIWA in Auckland.
Definitions
Glass sponge: unusual deep-water sponges which are highly siliceous. Their support skeletons resemble twisted ropes, fibre-glass sheets, woven baskets, or single huge spicules. They differ from other sponges in tissue organisation and symmetry of the spicules
Sclerosponges: sponges that have a calcium carbonate (aragonitic) base like a coral, with a living tissue veneer on the surface. Silica spicules are deposited in the calcium carbonate as the sponge grows outwards in rings.
Speleothems: stalactites or stalagmites, made from the precipitation of calcium carbonate from dripping water, deposited over time.
Spicules: The sponge skeleton is composed of a mineral skeleton of silicon dioxide (silica) or calcium carbonate (calcite) crystals known as spicules. These take amazing and beautiful forms and give structural support to the sponge.
Rock sponge: a sponge with a skeleton of silica so solid and interlocked that the sponge feels as hard as a rock (Water & Atmosphere 7(2): 11, and see Aquatic Biodiversity & Biosecurity 3: 3 for an article on rock sponges on New Zealand seamounts).
32Silicon dating
We estimate the age of different parts of sponges using 32Si dating. The method relies on the fact that a very small proportion of silicon found naturally in the sea is the radioactive isotope 32Si. (Most silicon (92.2%) has a molecular weight of 28 and in the marine environment the 32Si/28Si ratio is less than 1/1018.)
32Si is cosmically generated in the atmosphere and enters the marine environment in rain and snow and via runoff from rivers and streams. Once in the ocean 32Si behaves just like ordinary silicon and is incorporated into sponge silica as the sponge grows. When radioactive elements decay they give off radiation in the form of atomic particles or high-energy rays. We determine the amount of the radioactive element (in this case, Si) by measuring the amount of radiation emitted by that element.
32Si is the perfect tool for estimating the growth history of the large rock sponge shown on page 26 because it has a half-life of about 140 years. In other words, it will take 140 years for half of the 32Si originally incorporated into sponge silica to decay.
The detection of 32Si in sponge silica is very difficult because the atomic particle emitted is a very low energy one. However, when 32Si decays, it changes into phosphorus (32P). (This type of radioactive decay is known as ß- decay.) 32P is also radioactive and also undergoes ß- emission. The ß- particle emitted is a high energy one, thereby making its detection easier that its 32Si counterpart. The half-life of 32P is only about 14 days. So, detection of 32Si in the natural environment can be achieved by determining the ß- emission of the daughter isotope 32P.
Our radio-isotope analysis for this sponge study is conducted at Geological and Nuclear Sciences in Wellington, which has one of the few laboratories in the world capable of determining 32P activity.