PDF of this article (164 KB)
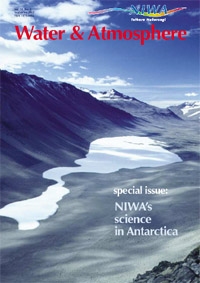
Ian Hawes, Anne-Maree Schwarz, Donna Sutherland and Clive Howard-Williams
Only a few kinds of aquatic organism can survive the extreme conditions in the streams and lakes of the Antarctic Dry Valleys.
The ice-free dry valleys adjacent to Antarctica’s McMurdo Sound have formed where the ice flowing outwards from the Polar Plateau of the continental interior is diverted away from a cluster of valleys by high inland mountain ranges. Instead of ice flowing from the plateau down these valleys, they are swept by strong, dry katabatic winds, which ensure that any precipitation falling as snow on the rocky valley floors is quickly lost to the atmosphere by the process of sublimation. The result is that the valleys are cold, dry deserts. The only ice that persists there is in the form of alpine glaciers along the steep valley sides and the permanent ice cover of the lakes on the valley floor.
When Captain Scott’s party of 1903 first discovered the dry valleys they considered them to be largely lifeless. We now know that in this apparently barren landscape a variety of aquatic ecosystems has developed, each with its characteristic flora and fauna. These systems include a network of ephemeral glacier meltstreams that drain into permanently ice-covered lakes. NIWA’s research programme in the dry valleys, in close collaboration with international colleagues (see McM–LTER panel below), is designed to find out how the extreme physical conditions structure the composition and activity of these remarkable inland aquatic ecosystems.

Of course, you only find aquatic life where there is liquid water and this is in short supply in a frozen continent. In the dry valleys the water supply is mainly from melting glacier ice, which in turn depends on a favourable combination of temperature, wind and sunshine. Ice will melt at air temperatures below 0 °C if it absorbs enough solar energy. So temperature and sunshine interact to determine the supply of water to streams. Just as climate varies from hour to hour, year to year, and century to century, the amount of water from glacier melt also varies over similar time scales. In some years the streams draining the glaciers may not flow at all; in others there may be veritable floods.
Glacier-melt streams
Only organisms that can survive and grow in the few days or weeks when liquid water is available in the melt streams, and can then tolerate the extreme winter conditions when everything is frozen at –50 °C, are capable of colonising shallow streams and ponds. So the stream communities usually comprise stresstolerant species of cyanobacteria, many of which are darkly pigmented for protection from the bright sunshine.
Without this protection, the high solar radiation (including UV) would be particularly damaging when they are exposed in dried or frozen states. Despite the harsh conditions, biomass and biodiversity of the microbial communities can be high. One reason is that there are no grazing invertebrates – or any other plants or animals – to compete with or eat the communities. So the high biomass is a result of slow accumulation over a long time, in the face of minimal disturbance.
Cyanobacteria can re-start photosynthesis within 48 hours of the return of liquid water after being “freeze-dried” in winter. Once re-hydrated they carry out the same functions as similar communities in less extreme environments. Low temperatures mean that metabolic processes happen slowly, yet the high biomass means that stream cyanobacteria can play a role in controlling the flux of nutrients along stream channels, and ultimately into the lakes. For example, microbial communities in the Onyx River that drains into Lake Vanda can remove up to 75% of the nitrate and phosphate that would otherwise reach the lake.
Ice-covered lakes
Growth conditions in the lakes are quite different from those in the streams. Here there is liquid water all year round, but the lakes retain an ice cover 3–5 m thick throughout the year. Perennial lake-ice is restricted to a few polar lakes, and it creates a special set of conditions. Ice cover prevents wind mixing, and hence dry valley lakes characteristically have exceptionally stable water columns. This allows such features as salt-water layers to persist for many years (see Lake levels below). It also limits the speed at which material such as nutrients, which tend to accumulate in the deep waters through sedimentation, can return to the upper layers of the lake. In addition, ice cover intercepts 80–99% of the sun’s energy at the surface of the lake, the exact value depending on the ice characteristics. The combination of low light, low temperature and slow supply of nutrients leads to very slow growth.
Much of the production appears to be associated with microbial mats (see also “Pond life on the McMurdo Ice Shelf”) on the beds of the lakes. As in the dry valley streams, the main organisms in these communities are cyanobacteria, but there are also diatoms, which are capable of very efficient use of the small amount of solar energy that penetrates the lake-ice cover. There are a few animals here too, though nothing more complex than tiny nematodes and tardigrades.

Diver sampling a microbial mat on the bed of Lake Vanda. (Photos: Ian Hawes)
Lake mats grow extremely slowly due to the low light but, like the communities in streams, they can accumulate large amounts of biomass because of minimal disturbance and an absence of grazing. A unique feature arising from the accumulated slow, undisturbed growth of the mats is their annual layering. These layers appear as alternate dark (winter) and light (summer) bands that offer a record of past changes that have occurred in the dry valleys. We have sectioned cores through the mats with enough resolution to identify the bands that were being laid down as Scott’s party trekked down the Taylor Valley a century ago.
The McMurdo Dry Valleys Long Term Ecological Research project (McM-LTER)
The long-term ecological research (LTER) network is an initiative funded by the US National Science Foundation to undertake fundamental ecological research in a wide range of the Earth’s ecosystems. By studying similar processes in a wide range of climate zones, a better understanding of the properties of those ecosystems will be possible than each study alone can provide. The McM-LTER, set in the heart of the McMurdo Dry Valleys, is an end-member in this set of ecosystems. A feature of LTER studies is that they include contributions from suitable research groups from around the world to fill gaps in expertise. NIWA currently collaborates with the McM-LTER by providing research on the ecosystem role of the microbial mats of the lakes. This highly fruitful collaboration allows us to share facilities and information, and adds value to both US and New Zealand-funded research programmes.
Lake levels – barometers of climate change

The effects of variable melt-water flow are seen in changes in lake levels. Levels change because these lakes occupy closed basins: they have inflows but no outflows. Hence the balance between evaporation/ sublimation from the lake surface and inflowing melt water sets the water levels. For example, old lake terraces on the side of the valley above Lake Vanda show that 5000 years ago the lake was at least 130 m deep. A little over 1200 years ago, the climate was such that inflows to Lake Vanda virtually dried up, and the lake evaporated to a brine pool just a few metres deep. Then the climate changed, and the inflow of melt water increased, and by the 1970s the lake depth was 65 m. Another slight change occurred in the 1980s when inflowing water volumes increased markedly, and the lake is now 75 m deep. The legacy of the drying period over 1000 years ago is still evident in the lake. When the melt water flowed in, it floated on top of the dense brine pool, and the hypersaline layer is still at the bottom of the lake today, as shown by measurements of conductivity at different lake depths.
Teachers: this article can be used for NCEA Achievement Standards in Biology (1.4, 1.7, 2.4, 2.5, 2.7, 3.2, 3.4), Geography (1.1, 1.6, 1.7, 2.1, 2.6, 2.7, 3.1, 3.6, 3.7). See other curriculum connections at www.niwa.co.nz/pubs/wa/resources
Ian Hawes and Anne-Maree Schwarz are based at NIWA in Hamilton; Donna Sutherland and Clive Howard-Williams are at NIWA in Christchurch.
This research receives FRST funding in the programme “Antarctic Aquatic Ecosystems” (C01X0220).