PDF of this article (86 KB)

Drew Lohrer
Small animals burrowing in the mud at the bottom of the sea could play a key role in increasing the productivity of our coastal waters.
Living organisms affect the physical, chemical, and biological aspects of their environment. The complex feedbacks between the environment and its inhabitants have become a major research focus in the last 10 years, as scientists have begun to probe the linkages between biodiversity and ecosystem functioning. One motivation for such research is to improve public awareness of the need to preserve biodiversity. If the loss of species changes the way an ecosystem works to the extent that benefits to humans are affected, then the importance of biodiversity becomes more tangible.
Each species in a community occupies a position in the food web and contributes to community dynamics. The loss of some species may significantly alter ecosystem processes. Key species are those whose presence and activities strongly influence the chemical or physical features of the habitat. For example, recent work has identified the native horse mussel (Atrina zelandica) as a key benthic species (see Water & Atmosphere 10(2): 22–23). Its large shells create physical structures and vertical relief in marine soft sediments. These provide points of attachment for sponges and soft corals as well as shelter from predators for small fish and invertebrates. Furthermore, the diversity of other animals tends to increase around Atrina beds because of sediment enrichment associated with horse mussel feeding.
Horse mussels are a common and conspicuous example of a key species. However, there are other key players in estuarine and coastal marine soft-sediment systems that are not so easily seen.
Heart urchins: nutrient distributors
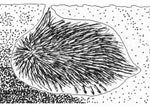
Heart urchins (Echinocardium australe) are common but cryptic animals living in the soft-sediment habitats of New Zealand. A scuba diver with a trained eye may observe a small ventilation slit in the surface of the sediment, or a characteristic track, but even these tell-tale signs are often missing. The sediment-coloured urchins, no more than 4 cm long, move with almost imperceptible slowness beneath the sediment surface.
Heart urchins ingest organic matter and microscopic plants as they move horizontally in the upper 3–4 cm of the sediment. As shown in the illustration, the movement of the animals displaces sediment particles, a process called bioturbation. This movement can disrupt the chemical gradients in the water within the sediments (known as pore water), thus influencing the transport of oxygen and nutrients across the sediment–water interface. The nutrients in sedimentary pore water are the fuel that supports the growth of microscopic algae both in the water (phytoplankton) and in the sediments (phytobenthos). The algae in turn are an important source of food for soft-sediment organisms and indeed the base of the entire marine food web.
Sediments on continental shelf areas (where burrowing urchins are widely distributed) can provide one-third to half of the nutrients in overlying waters. Therefore, these areas contribute significantly to marine primary productivity and support many of the world’s fisheries.
Measuring heart urchin effects
Using underwater time-lapse video recordings, combined with direct measurements in the field, we assessed the rates and patterns of Echinocardium movement. We found that the urchins generally move less than 1.5 m per day. However, given the large volume occupied by each urchin, a substantial amount of sediment is displaced by each individual. Furthermore, at densities of up to 70 urchins per square metre of seafloor, their collective effects are quite impressive. As much as 15 litres of sediment per square metre can be displaced each day.
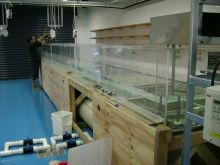
Because bioturbation can influence the transport of nutrients across the sediment–water interface, we performed field experiments to measure the ultimate effects of Echinocardium on the transport of nutrients between sediment and water. Using incubation chambers, we isolated square patches of seafloor along with about 25 litres of bottom water. Before fitting the chamber lids, we added or removed Echinocardium, so that there were treatments with 0, 4, 8, or 16 urchins per chamber. Then, by sampling the chamber water over time, we were able to relate changes in water chemistry to the density of Echinocardium.
Flux rates did depend on the density of Echinocardium inside the chambers. This was true for oxygen moving into sediments and for dissolved nutrients – phosphate, ammonium and nitrate – moving out into the water.
However, oxygen and nutrients are also used by organisms in the chambers, and this affects the concentrations that we can measure. Therefore, we also injected an inert chemical tracer into the chamber water at the start of the experiment. The inert tracer allowed us to estimate the amount of sediment mixed by Echinocardium movement, because the tracer penetrates faster and deeper into sediments that have been stirred up. The other water chemistry data showed the net effects of Echinocardium bioturbation, namely the provision of nutrients (phosphate, ammonium and nitrate) to the water column.
The feedbacks between urchin movement, nutrient efflux, and the growth of marine plants are still not well understood. However, with the data we have gathered so far, we can begin to model the linkages between water and sediment that are influenced by heart urchins. We aim to find out exactly what difference it would make if heart urchins were removed from the system. Since marine soft-sediment habitats support a broad array of desirable finfish and shellfish species and affect global elemental budgets (e.g., the carbon cycle), it is important to study the key species within them and the structural and functional roles they perform.
Teachers: this article can be used for NCEA Achievement Standards in Biology (2.5, 2.9, 3.2). See other curriculum connections at www.niwa.co.nz/pubs/wa/resources
Drew Lohrer is based at NIWA in Hamilton.