PDF of this article (1 MB)
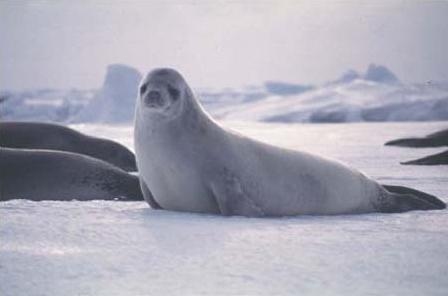
Murray Smith Craig Stevens Kim Currie Mike Harvey
Gas exchange at the sea surface is a vital link between the ocean and atmosphere, especially in relation to climate change. But measuring what is actually going on at the surface can be very difficult.
A crucial piece in the global warming jigsaw is the way greenhouse gases are transferred between air and sea. The oceans act as a remarkable sponge holding 50 times more carbon dioxide (CO2) than the atmosphere. Somewhere between 30 and 40% of the CO2 produced by human activity is thought to be absorbed by the world’s oceans. If we are going to predict the future climate, it is vital to determine where and how greenhouse gases are exchanged between the atmosphere and ocean.
The sea surface acts as a gas-transfer bottleneck governed by two key properties:
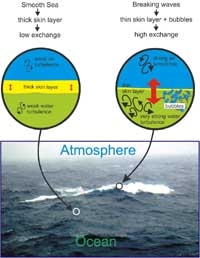
The mixing and bubbles created by waves and wave-breaking near the sea surface are crucial in determining the rate that gases and heat are exchanged between the atmosphere and ocean. Strong turbulence thins the diffusive skin layer and aids transport, allowing a more rapid exchange.
- The difference in gas concentration between air and sea. This controls the direction and rate at which the gas is moved. A larger difference pushes gas at a faster rate. The gas concentration in the water depends both on temperature and on biological processes (such as photosynthesis and respiration by the phytoplankton – tiny plants – which live in the water).
- The strength of ocean stirring processes. Stirring, or mixing, relieves the buildup of gas at the thin surface layer by transporting dissolved gas down to deeper water (see figure below left). Stirring is affected by waves and wave-breaking, which in turn are controlled by wind.
But it is extremely difficult to measure gas concentration differences and mixing because the ocean varies enormously over space and time, and so these properties vary too. Gas concentration difference can only really be determined by building up a map from repeated research voyages. The strength of the near-surface mixing is even harder to measure. Until now, simple models of gas transfer based on data from laboratory wind tunnels, lakes and other sources have been built around a single parameter – the wind speed. But exchange rates from these models vary by up to 100% from one another, indicating that other mechanisms are at play. Air bubbles pumped into the water by breaking waves are an obvious example. So we need a better understanding of what actually controls the ocean mixing. NIWA is tackling this problem by developing new ways of measuring the transfer of greenhouse gases between the atmosphere and ocean (see panel opposite).
Southern Ocean sponge
Teachers: this article can be used for Planet Earth and Beyond A.O 4 L7. See other curriculum connections at www.niwa.co.nz/pubs/wa/resources
Some regions of the ocean are more important than others in the transfer of gases between the air and the sea. The Southern Ocean, for example, has a special place in our understanding of the process. The cool waters have many of the right properties for gas absorption. In particular, localised plankton blooms there can use up a lot of CO2 in the water (see pages 28–30, this issue), thus increasing the air–sea concentration difference. Just as importantly, the stormy, wind-ravaged surface of the Southern Ocean makes it easier for gases to be absorbed by the ocean. It is quite likely that the transfer processes will increase markedly when measured in storm conditions. So, despite the surety of bad weather, the Southern Ocean often becomes our laboratory.
These transfer processes apply not only to CO2, but also to heat and to other climatically important gases.
Dimethylsulfide (DMS) is the most intriguing of these since it is produced in abundance by plankton processes in the ocean. Some scientists believe that when DMS escapes into the atmosphere it aids in the formation of clouds and changes their reflective properties by providing just the type of particles on which water will condense. This could counteract some of the warming effect caused by the CO2 increase. The possibility that microscopic life in the ocean could act as a climate regulator has fired the imagination of scientists who are now testing this theory. For more details see Water & Atmosphere 4(3): 9–12.
Future changes

In the Southern Ocean a vast area of large waves is built up in winter, shown in red. This will greatly assist the exchange of gases between air and sea. (Courtesy P. Challenor, Southampton Oceanography Centre, UK)
What can we say about future changes?
First, CO2 dissolves more easily in cold water than in warm water. So a warming ocean will store less CO2 than if it stayed cool. Changing wind and wave patterns will also change the balance between concentration gradients and mixing. Models predict higher rainfall over the Southern Ocean and reduced sea-ice coverage. These combine to produce fresher, lighter water that is less easily mixed by wind and wave action. The result could be a reduction in gas exchange at the ocean surface. Globally, the oceans will not be able to mitigate the effect of human production of CO2 as well as they do now. As the ocean temperature changes, phytoplank-ton types and numbers may also change, along with the amount of CO2 produced and used.
Unfortunately, the interacting exchange processes are complicated, and until they are better understood, we cannot be certain of the rate of future climate change. What we can say with certainty is that exchange with the oceans will play a key part in our global future.
Air–sea interaction tool box
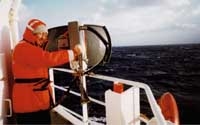
Using radar to measure waves from RV Tangaroa on a rare calm day in the Southern Ocean.
Reliable instruments for measuring many ocean properties are now readily available from commercial companies. However, few tools can measure very close to the surface, where there are large, violent waves. Measuring these waves and their breaking properties is challenging. A novel tool developed at NIWA is a kind of radar that has more in common with a police speed trap than a normal ship radar. It sends out a thin beam of electromagnetic energy that spreads across the water surface over distances of a few hundred metres. This allows us to make space–time maps of the waves and, crucially, where they are breaking.
Another instrument we use to measure mixing in the ocean is a microstructure temperature profiler. It sinks down a few metres then rises back to the surface, measuring the temperature every millimetre along the way. This tells us how turbulent the upper few metres of the water column are, and the capacity to carry dissolved greenhouse gases from or to the surface layer. We are presently developing a larger version that works in the same way in the open ocean under all kinds of sea conditions.
Carbon dioxide is measured while the research ship is under-way. A continuous flow of seawater is sprayed into a chamber where CO2 can escape from the water into the air within the container. The concentration of CO2 is then measured by the relative absorption of an infrared light beam as it passes through the gas sample. The solubility of CO2 in the ocean is very sensitive to temperature, so temperature must be measured carefully throughout the process.
A direct tool for measuring gas exchange in the air just above the ocean surface is a method called Relaxed Eddy Accumulation. This can separate air being carried up from that carried down by turbulent eddies in the air. The up-and-down draft samples are then analysed by highly sensitive gas-detecting devices to determine the net transport of gas at the surface. This technique has recently been employed very successfully on land (see Water & Atmosphere 8(3): 5). We are now developing methods of working at sea when all the instruments are surging up and down in the unforgiving Southern Ocean swell!
Murray Smith, Craig Stevens and Mike Harvey are all based at NIWA in Wellington; Kim Currie is at NIWA in Dunedin.