PDF of this article (6 MB)
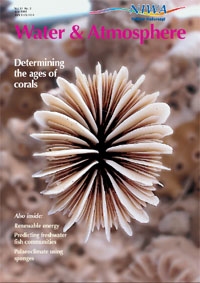
Alistair Dunn Neil Andrew
Computer modelling of apparently simple interactions in a reef environment has shown that these systems can change in a surprisingly complex way.

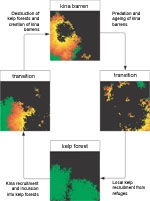
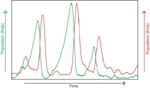
When it comes to biodiversity, reefs (ridges of rock, sand, coral or other hard materials lying at or near the surface of the ocean) are often thought of as stable systems unless catastrophic events such as storms or floods intervene. We imagine the same sorts of organisms inhabiting these places, year in, year out. However, the reality is more complex. There appear to be long-term changes in the ecology of rocky reefs that cannot be explained by simple processes alone.
Scientists can study these changes in various ways, including gathering long-term data, field experiments, and theoretical studies. Almost all the science on rocky reef ecology in New Zealand has been field-based and empirical, and the power of computer modelling to contribute to a better understanding of these systems has been under-used. However, recent work at NIWA using computer simulations run over long periods has produced some surprising outcomes.
Computer simulations to describe reef systems
Theoretical studies use simulation models, which define the reef as an array of identically programmed cells or blocks that can interact with one another. The way the cells interact is defined by so-called transition rules. Because we are trying to describe an ecological system, each cell can represent, for example, the number of organisms and the presence of different species at each location.
Such methods have been used to try to understand and/or predict many ecological phenomena. These include starfish outbreaks, patch dynamics of seaweeds and limpets on rocky shores, competitive growth between marine species, the spread of invasive species, and the behaviour of fish schools in the presence of predators.
The recent NIWA work suggests that reef biodiversity is a very complex but chaotic system. Because reefs are chaotic, individual populations – and therefore local biodiversity – can be extremely variable. By defining simple rules for the interaction of individual species, a complicated system of population fluctuations can result.
Simple rules, complex system
Our reef simulation work focuses on the cycles in community state on shallow subtidal reefs associated with changes in the abundance of kina – a kind of sea urchin (echinoderm). High densities of kina remove large brown kelp from reefs, and this leads to changes in the types and number of fish and invertebrates –including some of commercial value. The processes that regulate the number of kina are therefore critical to our understanding of community structure.
In our current project we are investigating how simple, local, transition rules can be devised to generate scenarios that mimic simplified, but real, ecological systems. This involves constructing a simple predator–prey model, where the incidence and spread of kina relies on the amount of kelp in the local neighbourhood. Similarly, the incidence and spread of kelp is modified by the amount of kina. The simulation is run over many time cycles, and uses stochastic rules for species spread and species recruitment.
High variation for new stable state
The unexpected result from our simulations is that simple rules often translate into highly dynamic population changes. Modelling results suggest that high variation in population densities over time could be the natural state for systems driven by local transition rules.
The inclusion of a space factor has been a central part of our models. Many biological processes are influenced by what is happening in adjacent areas. For example, the level of kelp recruitment depends on how much is growing close by, and the probability of being grazed by kina depends on populations moving in from other areas. Overall, our simulations are at an early stage, but it is clear that seemingly simple relationships between species can lead to complex community responses when viewed over a long time. Understanding the dynamics of population change for key species is critical to managing impacts on natural systems such as reefs and protecting biodiversity.
Teachers: this article can be used for NCEA Achievement Standards in Biology (1.2, 2.2, 2.5, 3.4). See other curriculum connections at www.niwa.co.nz/pubs/wa/resources
Alistair Dunn and Neil Andrew are based at NIWA in Wellington.
Definitions
Empirical: relying on or derived from observation or experiment.
Stochastic: involving a random process, variable, or variables, i.e., involving chance or probability.
Acknowledgments
This project is part of the FRST-funded programme “Sustainability and Enhancement of Coastal Reef Fisheries of Economic and Cultural Importance” (C01X0209).